Matthew P. Wahl, Rebecca L. Scalzo, Judith G. Regensteiner, Jane E. B. Reusch
Перевод из журнала Frontiers in Endocrinology (оригинал).
Аннотация
За последние десятилетия сахарный диабет приобрел характер одного из самых распространенных заболеваний не только в США, но и во всем мире. По последним подсчетам, количество пациентов в США насчитывает 30,3 млн, а во всем мире уже более 422 млн человек. Сахарный диабет 2 типа ассоциирован с высоким сердечно-сосудистым риском, что является ведущей причиной смерти у больных СД. В то время как приверженность физическим упражнениям – один из базовых методов лечения СД, в литературе хорошо описаны нежелательные последствия аэробных нагрузок, что влечет за собой создание дополнительного барьера для пациентов, пытающихся ввести в свой образ жизни регулярные физические нагрузки. Физиологические механизмы, обусловливающие ухудшение состояния при выполнении физических упражнений, включают инсулинорезистентность, патологические изменения сердца, перестройку работы митохондриального аппарата и обеспеченность кислородом. В этой статье обсуждаются проблемы, связанные с физической активностью у больных СД, а также потенциальные терапевтические подходы к их решению.
Масштаб проблемы
Распространенность СД в США крайне быстро увеличивается (с 1998 по 2010 заболеваемость выросла на 75%) (1). Количество больных в США составляет 30,3 млн человек. СД 2 типа ассоциирован с высоким сердечно-сосудистым риском, что является ведущей причиной смерти у взрослых больных данной группы (2-4). В 2015 году СД занимал седьмое место в списке лидирующих по смертности заболеваний в США (2). Общая сумма прямых и косвенных расходов, связанных с СД, превышает 1,3 триллиона долларов и эта цифра не окончательная, так как в ближайшее время предполагается ее заметный рост (5,6).
В Стандартах помощи больным СД, разработанных в 2018 году Американской Диабетической Ассоциацией, для большинства больных СД 1 и 2 типов рекомендованное количество физической нагрузки за неделю должно составлять 150 или более минут физической активности умеренной или высокой степени интенсивности, а также два занятия, включающие силовые упражнения (7). Несмотря на хорошо известное положительное влияние физических упражнений, только 51% взрослого населения США следует рекомендациям по части аэробной физической нагрузки (8). Занятия спортом связывают не только с улучшенным контролем веса пациента, но также и со снижением артериального давления, увеличением чувствительности тканей к инсулину, улучшением контроля уровня глюкозы и нормализацией липидного профиля (9). Большинство последних исследований доказывают, что умеренная или высокая аэробная нагрузка связана со значительным снижением сердечно-сосудистого и общего риска смертности у больных СД 1 и 2 типов (10). Напротив, отсутствие физической активности или сидячий образ жизни, как известно, оказывают негативное воздействие (11,12). Целью данного обзора является описание последних достижений в понимании взаимосвязи между СД и проблемами, которые могут возникнуть при занятиях спортом.
Клиническое значение
Пациенты с СД ограничены в физической активности и имеют пониженный уровень кардиореспираторной выносливости (КРВ). Иначе говоря, их способность к максимальному потреблению кислорода (максимальная аэробная способность, VO2 max) снижена примерно на 20% по сравнению с нормой (20-25). Это крайне важный показатель, так как его снижение статистически значимо влияет на увеличение показателя сердечно-сосудистой смертности (11, 20, 22, 26-28). Вторым аспектом, ограничивающим способность переносить физические нагрузки, является то, что пациенты с СД 2 типа имеют более медленную скорость поглощения кислорода тканями во время длительных нагрузок (21, 29). Это приводит к снижению способности организма адаптироваться к резкому увеличению потребности кислорода в тканях при выполнении упражнений.
Большинство исследователей связывают снижение КРВ при СД 2 типа с инсулинорезистентностью, эндотелиальной дисфункцией, недостаточной перфузией миокарда во время упражнений, патологически высоким ДЗЛА (давлением заклинивания легочной артерии), замедленным кровоснабжением верхних и нижних конечностей, а также нарушением в работе митохондриального аппарата скелетных мышц (20-24, 29, 31-36). Нарушение уровня КРВ наблюдается как у детей, так и у взрослых, даже при условии недавно поставленного диагноза СД2 и отсутствии явных признаков патологии сердечно-сосудистой системы (23, 24). Снижение КРВ, по-видимому, не зависит от последствий ожирения или сниженной повседневной физической активности (19-24, 37-41). Из-за методологических ограничений на сегодняшний день нам не хватает знаний о том, как каждая из патологий способствует снижению КРВ. К тому же, недостаток информации о том, какие из осложнений потенциально обратимы, ограничивает круг клинически значимых выводов. Целью данного обзора является обсуждение основных механизмов снижения КРВ при СД2, обозначение новых данных, сообщающих о вкладе микроциркуляторных нарушений в проблему сниженной толерантности к физическим нагрузкам у пациентов с СД2.
Физические упражнения как метод лечения СД2
Учитывая значимость такого параметра как КРВ для тренировок у пациентов с диабетом, очень важно охарактеризовать физиологические механизмы сниженной толерантности к физическим нагрузкам и обозначить конкретные терапевтические стратегии для увеличения КРВ. Известно, что занятия спортом способны предотвратить развитие СД2 даже при отсутствии снижения веса в результате тренировок (42). Многие исследования на сегодняшний день сообщают о том, что тренировочный процесс сам по себе со временем увеличивает способность пациентов переносить физические нагрузки (20-22, 29, 43-49). Однако показатель снижения смертности у больных СД2 остается под вопросом. Например, исследование Look Ahead не показало значимого увеличения выживаемости у групп с модифицированным образом жизни или регулярной физической активностью (50). В этом исследовании у пациентов был низкий риск неблагоприятных исходов (отчасти из-за общего снижения смертности от сердечно-сосудистых заболеваний у людей с СД, вероятно, связанных с использованием улучшенной модификации факторов риска сердечно-сосудистых заболеваний при проведении статистического анализа). Тем не менее, исследование Look Ahead также показало, что изменение образа жизни у пациентов с СД2 способствует улучшению качества жизни, снижению затрат на медикаменты и уменьшению количества необходимой сахароснижающей терапии в группе, максимально изменившей привычный образ жизни (51). Ранее было показано, что несмотря на увеличение показателя КРВ после физических нагрузок, непереносимость последних остается неизменной у пациентов с СД2, в отличие от людей с аналогичной физической подготовкой без СД2, что, возможно, объясняется сочетанием модифицируемых и необратимых нарушений (21, таблица).
Оценка потенциальных механизмов непереносимости физической нагрузки при СД2
Предыдущее исследование, проведенное в нашей лаборатории, продемонстрировало прямую зависимость между чувствительностью к инсулину и VO2 max (20, 31). С целью доказать эту теорию мы решили исследовать, обладают ли средства, увеличивающие чувствительность тканей к инсулину, способностью улучшать толерантность к физической нагрузке. Наша группа исследовала эффекты розиглитазона (РГЗ) из класса тиазолиндионов (ТЗД) на способность группы пациентов переносить активную физическую нагрузку (31). ТЗД увеличивают чувствительность к инсулину скелетных мышц и жировой ткани. В результате этого исследования наблюдался значительный прирост VO2 max (на 7%) у участников с СД2, которые в течение 4 мес. получали терапию розиглитозоном (4 мг/сут) (31). Чувствительность к инсулину, определенная с помощью индекса HOMA, и состояние эндотелия сосудов, оцененное с помощью УЗДГ брахиоцефальных артерий, были значительно улучшены у группы, получавшей терапию. Вклад увеличения чувствительности тканей к инсулину под действием ТЗД в переносимость физической нагрузки остается неясным из-за большого количества факторов, влияющих на КРВ.
Результаты, полученные в ходе этого исследования, были воспроизведены Kadoglou et al. (52). В их исследовании продемонстрированы дополнительные плюсы сочетания физических упражнений с приемом ТЗД (52), что также было подтверждено на животной модели (53). Влияние ТЗД на возможность выполнения активных физических упражнений, по-видимому, различается в зависимости от статуса заболевания, так как пациенты с СД2 и сопутствующими ССЗ не показали роста VO2 max (54). Результаты вышеуказанных работ подтверждают, что снижение инсулинорезистентности положительно влияет на показатель VO2 max при неосложненном СД2. В настоящее время в США розиглитазон реже используется как средство в терапии СД2, отчасти из-за опасений, что он увеличивает риск ССО (сердечно-сосудистые осложнения) (утверждение оспаривается FDA (55)), поэтому могут потребоваться дальнейшие исследования с другими препаратами, увеличивающими чувствительность к инсулину.
В отношении КРВ были исследованы эффекты еще одного известного препарата – метформина. Его сахароснижающее действие обусловлено стимулированием АМФ-активируемой протеинкиназы (АМФК). Исследование, опубликованное в 2008 году Braun et al., показало, что прием метформина здоровой группой привело к уменьшению VO2 max на 2,7% по сравнению с группой контроля (56). Похожее исследование на пациентах с инсулинорезистентностью (по HOMA) также продемонстрировало значительное снижение VO2 (пиковое) на метформине, который группа испытуемых принимала в течении 3 месяцев (57). Несмотря на такие неутешительные выводы, влияние метформина на VO2 (пиковое) не определялось на людях с подтвержденным СД2. Отдельные исследования касались ответа на метформин у людей с метаболическим синдромом, но их данные разнятся в отношении корреляции КРВ с приемом метформина. В одном из недавних исследований было показано значительное снижение VO2 max у пациентов с метаболическим синдромом при приеме метформина в течении 6 недель (58). В то же время, исследования влияния метформина на пациентов с преддиабетом не показали значимого изменения КРВ при ведении группы без сопутствующих лечению тренировок, однако не было замечено и негативного его влияния на VO2 max при проведении пробной тренировки с активной физической нагрузкой (59, 60). Как сообщается, метформин способен ингибировать I комплекс в дыхательной цепи переноса электронов (61, 62). На основании этого было высказано предположение о том, что метформин может снижать КРВ у пациентов с диабетом. Так как метформин является препаратом первой линии для терапии СД2, необходимы дальнейшие исследования для определения влияния этого широко распространенного препарата на КРВ в популяции, где он назначается.
Эпигенетические механизмы также играют роль в патогенезе СД2 (63). Имеются исследования, показывающие, что у данной группы больных высокая степень физической нагрузки приводит к гипометилированию ДНК в промоторных областях скелетных мышц (64). В литературе есть предположения, что некоторые заболевания, в частности диабет, могут влиять на эти эпигенетические реакции (65). Это область активной работы исследователей под эгидой NIH Common Fund Molecular Transducers of Physical Activity Program. Как только механизмы этих изменений в здоровой популяции станут понятны, исследователи смогут выяснить, существуют ли различия в адаптивном ответе в контексте диабета.
Митохондриальная дисфункция
Сахарный диабет ассоциирован с дисфункцией митохондриального аппарата миокарда и скелетных мышц (66, 67). Есть данные о том, что также могут быть задействованы и другие мышечные ткани, так как при СД нарушается функция митохондрий гладких мышц сосудистой стенки (68). Эти находки вполне объяснимы тем, что нефункциональная митохондрия способствует снижению моторики сосудов и возникновению избытка активных форм кислорода (АФК) (69,70). Сосудистые АФК ответственны за развитие местной воспалительной реакции и снижение эластичности сосудистой стенки – предвестников развития сердечно-сосудистой патологии (71). Исследования на здоровых субъектах без диабета показывают, что физические упражнения улучшают состояние митохондрий скелетных мышц и сосудов, а также препятствуют их повреждению (72-76). Но в ходе исследования было обнаружено, что у крыс с артериальной гипертензией или метаболическим синдромом и неосложненным СД2 не наблюдалось никакого положительного ответа митохондриального аппарата (изучалась аорта) после проведения тренировок (68). Несостоятельность адаптивного механизма митохондрий у крыс с диабетом была неожиданной для исследователей и навела на мысль о необходимости рассмотреть митохондриальный аппарат сосудов как новую терапевтическую мишень при СД2.
Для того, чтобы определить митохондриальную дисфункцию как мишень для терапии исследовали связи между патологией сосудов при СД2 и известными митохондриальными регуляторными путями. Эндотелиальная дисфункция является частой сосудистой патологией при диабете и, как известно, ее развитие частично находится под контролем эндотелиальной синтазы оксида азота (eNOS) (77). Исследование группы Nisoli’s установило, что оксид азота (NO) является одним из главных регуляторов биогенеза митохондрий в различных тканях (78). Исходя из этого были исследованы эффекты, которые ингибирование eNOS может оказывать на биогенез митохондрий в сосудах. В публикации 2013 года отмечено, что ингибирование eNOS блокировало митохондриальную адаптацию к физической нагрузке в аорте крыс (79), подтверждая важную роль NO в биогенезе митохондрий сосудов и роль этого вещества на процесс адаптивной реакции к физической нагрузке.
Дисфункция поджелудочной железы
Начало тренировки у людей без диабета сопряжено со снижением секреции инсулина и повышением уровня глюкагона (80-82). В ранее сделанных сообщениях указывается, что упражнения выполняют своеобразную “трофическую” функцию панкреатических бета-клеток (83, 84). К сожалению, исследования сочетания дисфункции поджелудочной железы с физической нагрузкой не проводились. Дисфункция и недостаточность бета-клеток поджелудочной железы играют ключевую роль в патогенезе сахарного диабета. Процесс старения организма может приводить к нарушению углеводного обмена как за счет повышения резистентности к инсулину, так и нарушения его секреции (85-89). Было показано, что есть определенные механизмы, ответственные за снижение секреции инсулина у возрастных пациентов. К этим механизмам относят сниженную экспрессию β(2)-адренорецепторов (90), снижение активности в работе внутриклеточных Ca-зависимых сигнальных путей (91) и хронический оксидативный стресс (92). Следовательно, вполне вероятно, что процесс старения у людей с диабетом может быть напрямую связан с патологическим соотношением глюкагона к инсулину, что влияет на способность этих пациентов переносить физическую нагрузку (93). Также следует отметить, что митохондрии β-клеток играют важную роль в секреции инсулина. Дисфункция митохондрий в β-клетках ведет к недостаточной секреции инсулина, а затем и к нарушению секреции глюкагона (94-96). Этот предполагаемый патологический механизм получил дальнейшее подтверждение в недавнем исследовании, изучавшем негативное воздействие такролимуса на митохондрии β-клеток, что впоследствии привело к недостаточности бета-клеток поджелудочной железы (97). Нарушение в работе митохондрий, отмеченное у возрастных пациентов и людей с диабетом, может негативно сказываться на уровне «метаболической гибкости» митохондриального аппарата, которая необходима для быстрой адаптации клеток поджелудочной железы к метаболическим изменениям при физической нагрузке. Оценка реакции островков Лангерганса на физическую нагрузку у людей с неосложненным диабетом является открытой областью для будущих исследований.
Дисфункция NOS как терапевтическая мишень
Чтобы определить, возможно ли препятствовать ингибированию NOS и улучшить адаптивный ответ на физическую нагрузку, исследователи обратили внимание на класс инкретинов, стимулирующих секрецию инсулина: агонистам глюкагоноподобного пептидного рецептора 1 (АР ГПП-1) и ингибиторам дипептидилпептидазы 4 (иДПП-4). ГПП-1 действуют через рецепторы, связанные с G-белками, которые в большом количестве представлены в сосудистой стенке и, как было показано, стимулируют eNOS и увеличивают синтез циклического АМФ (цАМФ), тем самым приводя к улучшению эндотелиальной функции и увеличению тканевой перфузии, а также способствуют более эффективному использованию глюкозы мышцами (98-101). Чтобы изучить эффекты инкретинов на переносимость физической нагрузки, состояние эндотелия и митохондриальную адаптацию, было изучено влияние саксаглиптина (иДПП-4) на крысах Goto-Kakazaki (модель крысиного инсулинорезистентного СД). Саксаглиптин в комбинации с физическими нагрузками выявил стимулирование eNOS и восстановление активного митохондриального биогенеза в сосудистой стенке (70). В более раннем исследовании была протестирована группа людей с неосложненным СД2, где все пациенты получали АР ГПП-1 эксенатид (102). Лечение эксенатидом без сопутствующих физических нагрузок привело к улучшению как диастолической функции сердца, так и эластичности аорты, но не увеличило VO2 max и не повлияло на эндотелиальную функцию (была исследована с помощью поток-опосредованной дилатации) (102). Эти данные позволяют предположить, что отдельное вмешательство в виде лекарственной терапии (без сопутствующих тренировок) в некоторой степени положительно влияет на сердечную, сосудистую и мышечную функцию (последнее не оценивалось в этом исследовании). В исследовании не изучалось влияние эксенатида на eNOS и NO. Вполне возможно, что дисфункция eNOS сделала объекты исследования устойчивыми к регуляции синтеза NO с помощью цАМФ, либо же комбинация АР ГПП-1 и тренировок необходима для достижения адаптивного ответа и изменения VO2 max. Эти наблюдения позволяют предположить, что синтез NO может иметь решающее значение в процессе ответа сосудов на физическую нагрузку. В настоящее время изучаются и другие методы, направленные на преодоление дисфункции NOS у пациентов с диабетом.
Другие агенты с механизмом действия, основанным на увеличении чувствительности к инсулину, были также исследованы в качестве средств для увеличения толерантности к физическим нагрузкам. Группу составляли животные модели без диабета, а затем здоровые люди. Изучалось влияние стимуляции 5’АМФ-активируемой протеинкиназы (АМФК) и применение лигандов к рецепторам, активирующим пролиферацию пероксисом (PPAR) типа дельта. В исследовании 2008 года прием перорального активного агониста АМФК (AICAR) в течение 4 недель привело к увеличению физической выносливости на 44% у лабораторных мышей (103). В исследовании 2017 года лабораторные мыши, которые получали терапию лигандами к PPAR типа дельта, могли “работать” примерно на 100 минут дольше (на 70% больше по сравнению с контрольной группой мышей) (104). Другие исследования диабетических моделей с использованием этих агентов проводятся, но на данный момент не завершены.
Ингибиторы НГЛТ 2 типа являются относительно новым классом препаратов с доказанной эффективностью и значимым влиянием на вероятность ССО (сердечно-сосудистых осложнений) и смертность больных с диабетом (105, 106). Недавно было обнаружено, что ингибиторы НГЛТ 2 типа увеличивают чувствительность к инсулину скелетных мышц у крыс с диабетом (107), а также жировой ткани эпикарда человека ex vivo (108). В исследовании Li et al. ингибирование НГЛТ с помощью флоризина заметно снижало эндотелиальную дисфункцию путем увеличения уровня синтеза NO в эндотелиальных клетках пупочной вены человека (109). К сожалению, пока что нет опубликованных исследований, которые изучали бы связь ингибирования НГЛТ 2 типа и КРВ, за исключением явного положительного влияния препаратов данной группы в контексте сердечной недостаточности (105, 106, 110). В свете последних публикаций, сообщающих о значительном улучшении сердечной функции, снижении ригидности аорты и роста почечной перфузии у исследуемых групп пациентов, вполне вероятно, что ингибиторы НГЛТ 2 типа могут оказывать действие и на патофизиологические механизмы, влияющие на толерантность к физическим нагрузкам при СД2 (111-116). Необходимы дальнейшие исследования, которые смогут оценить влияние ингибиторов НГЛТ 2 типа на чувствительность тканей к инсулину, синтез NO и переносимость физической нагрузки.
Мышечная микрососудистая перфузия
Мы обсудили роль NOS в адаптации сосудов к стрессу и физической нагрузке. Тем не менее, все еще неизвестно, каковы последствия снижения активности NOS для скелетных мышц и функции сердца. Возникает вопрос, ответственны ли изоформы NOS скелетных мышц и сердца (eNOS и нейрональные NOS, nNOS) за адаптацию к тренировкам или все дело в NOS-опосредованном действии на сосуды и кровоток. McConnell сообщает о том, что митохондриальная адаптация скелетных мышц не зависит от присутствия eNOS или nNOS (117). Другое недавнее исследование указывает на то, что изменения микрососудистого мышечного кровотока необходимы для регуляции эффекта инсулина на ткани (118). В параллельном исследовании Liu и Barrett также отмечают, что процесс воздействия инсулина на скелетные мышцы находится под влиянием NOS (119, 120). Обобщая результаты, можно заключить, что эти исследования подтверждают предполагаемую модель, в которой корректное действие инсулина на скелетную мускулатуру включает в себя координацию доставки инсулина, зависимую от кровотока, с корректной передачей сигнала в скелетной мышце.
Подобно взаимосвязи между сосудистой перфузией в мышцах и действием инсулина, перфузия сердечной и скелетной мышц имеет решающее значение для адаптации организма во время тренировки. В одном из недавних исследований было родемонстрировано снижение перфузии миокарда (визуализация осуществлялась путем МИБИ сканирования) во время тренировок у людей с СД2, что было ожидаемо, учитывая наличие патологической перфузии тканей, связанной с развитием сердечной дисфункции при СД2 (19). Кроме того, сообщалось о замедлении кровотока в скелетных мышцах в начале тренировки у людей с СД2 (22). Говоря о митохондриальной дисфункции скелетных мышц, в настоящее время не решены несколько ключевых вопросов: 1. Какова взаимосвязь между окислительной способностью скелетных мышц in vivo и VO2 max? 2. Каков вклад нарушения мышечной перфузии в снижение окислительной способности скелетных мышц? 3. Играет ли роль полноценный ответ организма на тренировку или же ключевым звеном является процесс активации скелетной мускулатуры? Ограничение и гетерогенность перфузии скелетных мышц из-за повреждения капилляров могут вносить вклад в лимитирование процессов окислительного фосфорилирования в мышечной ткани у пациентов с СД2, и, в конечном счете, приводить к снижению КРВ. В доказательство этой модели было опубликовано сообщение о том, что по результатам исследования группа людей с СД2 имела такой же набор капилляров, что и здоровые субъекты, но при этом во время упражнений был выявлен сниженный уровень потребления оксигемоглобина по сравнению с людьми без диабета (121). Исходя из проведенных работ, можно с уверенностью сказать, что в основе патологического снижения окислительной способности скелетных мышц лежит как митохондриальная дисфункция, так и нарушение перфузии мышечной ткани, включая неравномерное распределение кровотока.
В дополнение к представлению о патогенезе низкого уровня перфузии мышечной ткани при диабете группой Jefferson Frisbee проводился анализ плотности капилляров, степени перфузии и их связи с развитием мышечной слабости (122). Этот анализ включал теоретическое моделирование распределения кровотока и прогнозируемой доставки кислорода в мышечное волокно. Экспериментальные данные были получены при исследовании худой крысы Цукера и крысы Цукера с ожирением и метаболическим синдромом. Целью являлось выяснить, что именно вносит вклад в снижение мышечного VO2 при метаболическом синдроме. Мышечный кровоток у больной крысы был ниже, а вот уровень кислорода венозной крови был выше. Проще говоря, несмотря на меньшую подачу кровотока в мышцу, для выполнения той же работы потребовалось меньше кислорода. Возможными объяснениями этого могут быть сниженная плотность капилляров и/или снижение эффективности капиллярной перфузии. У изучаемой модели наблюдалось связанное с диабетом снижение плотности капилляров, но, по сравнению со здоровой моделью, эта разница составила лишь 20%. Другой стороной проблемы в дефекте потребления кислорода мышцами являлось неравномерное распределение кровотока (а именно, эритроцитов). Исследователи смогли подтвердить, что модель была воспроизведена верно, использовав антиоксидант TEMPOL, после чего восстановилось примерно 80% кровотока (123). Такой ответ не был бы возможен, если бы дефект был вызван фиксированным повреждением, таким, как снижение плотности капилляров. Полученные результаты показывают, что несоответствие в потреблении кислорода мышцами (VO2 в мышцах) в первую очередь объясняется неоднородностью перфузии мышечной ткани (122). Значимый рост потребления кислорода мышцами, достигнутый с помощью антиоксиданта TEMPOL, дал понять, что неоднородность микрососудистой перфузии представляет собой неизученную терапевтическую мишень для достижения физиологической оксигенации тканей и нормальной мышечной функции у больных с диабетом.
Подводя итог, можно сказать, что нарушение мышечной перфузии является вероятным фактором снижения уровня VO2 и КРВ при диабете. Последние данные, полученные на людях и животных моделях с использованием антиоксиданта TEMPOL и других препаратов, показывают, что нарушение перфузии мышц поддается коррекции (123). Улучшение мышечного кровотока является новым терапевтическим направлением для увеличения КРВ и эффективной адаптации к физическим нагрузкам при диабете. Последующие исследования должны быть сосредоточены на влиянии вмешательств, нацеленных на кровоток в сердечной и скелетной мышцах, а также на изучение взаимодействия уровня перфузии и толерантности к физическим нагрузкам.
Сердечная функция
Инвазивные и неинвазивные методы исследования состояния сердечно-сосудистой системы у больных СД2 разных возрастных групп во время физической нагрузки демонстрируют наличие патологических изменений у большинства пациентов (23, 24). В одном из исследований была проведена катетеризация правых отделов сердца с целью исследовать сердечную функцию во время тренировки у группы женщин с пременопаузой, ведущих малоподвижный образ жизни и страдающих избыточным весом (с неосложненным СД2 и без него). При сравнении двух групп не было обнаружено различий в значениях сердечного выброса в покое и во время тренировки (19). Тем не менее, наблюдалось значительное увеличение ДЗЛА во время физической нагрузки в группе с СД2, что указывает на возможность снижения сократительной способности желудочков. Увеличение ДЗЛА коррелировало со снижением перфузии миокарда, которая оценивалась с использованием МИБИ (метоксиизобутилизонитрил) сканирования во время стресс-теста (19). Подобная зависимость наблюдалась у подростков с диабетом и без него при использовании неинвазивных методов исследования (23, 24). Необходимо более четкое понимание отдаленных последствий этих субклинических изменений. Кроме того, неизвестно, будут ли агенты, улучшающие перфузию скелетных мышц, оказывать такое же влияние на перфузию миокарда. Взаимосвязь между диастолической дисфункцией, повышенным ДЗЛА и переносимой нагрузкой может объяснять влияние сердечной дисфункции на КРВ у пациентов с СД2.
Выводы
Даже пациенты с недавно диагностированным диабетом имеют сниженную толерантность к физической нагрузке, о чем свидетельствует низкий уровень КРВ при максимальной и субмаксимальной нагрузке по сравнению с аналогичными пациентами с избыточным весом и сидячим образом жизни без диабета. Несмотря на улучшение показателей КРВ в ходе систематических тренировок, нарушение переносимости физической нагрузки сохраняется у людей с СД2 в отличие от людей без диабета по не до конца ясным причинам. Наличие сахарного диабета может влиять на с развитие патологических процессов в сердечно-сосудистой и мышечной системах под действием активной физической нагрузки (рисунок 1). Поэтому можно заключить, что проблема является многофакторной, что исключает возможность единого терапевтического подхода к решению проблемы. Таким образом, методы повышения толерантности к физическим нагрузкам при СД2 должны быть мультифакториальными и ориентироваться на участников, у которых предварительно были в комплексе оценены параметры сердечно-сосудистой системы и скелетной мускулатуры.
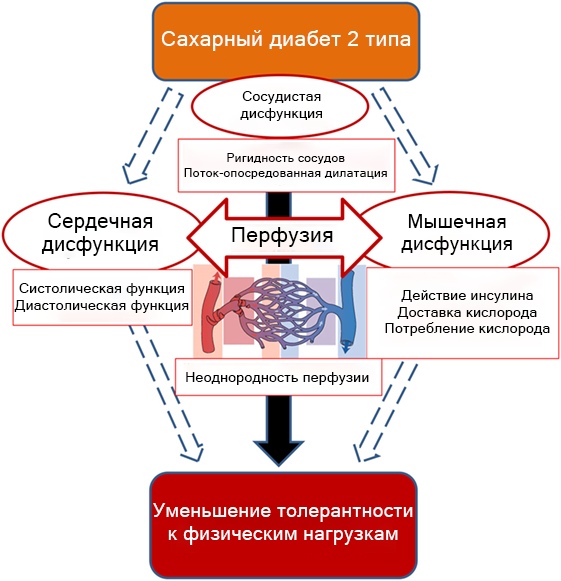
Рисунок 1. При СД2 повреждаются сердечные, макроваскулярные и микроваскулярные функции, что коррелирует со снижением КВС.
Литература
1. Cheng YJ, Imperatore G, Geiss LS, Wang J, Saydah SH, Cowie CC, et al. Secular changes in the age-specific prevalence of diabetes among U.S. adults: 1988–2010. Diabetes Care (2013) 36(9):2690–6. doi:10.2337/dc12-2074
PubMed Abstract | CrossRef Full Text | Google Scholar
2. Centers for Disease Control and Prevention. National Diabetes Statistics Report, 2017. Atlanta, GA: Centers for Disease Control and Prevention, US Department of Health and Human Services (2017).
3. Haffner SM, Lehto S, Ronnemaa T, Pyorala K, Laakso M. Mortality from coronary heart disease in subjects with type 2 diabetes and in nondiabetic subjects with and without prior myocardial infarction. N Engl J Med (1998) 339(4):229–34. doi:10.1056/NEJM199807233390404
PubMed Abstract | CrossRef Full Text | Google Scholar
4. Emerging Risk Factors CollaborationSarwar N, Gao P, Seshasai SR, Gobin R, Kaptoge S, et al. Diabetes mellitus, fasting blood glucose concentration, and risk of vascular disease: a collaborative meta-analysis of 102 prospective studies. Lancet (2010) 375(9733):2215–22. doi:10.1016/S0140-6736(10)60484-9
PubMed Abstract | CrossRef Full Text | Google Scholar
5. Bommer C, Heesemann E, Sagalova V, Manne-Goehler J, Atun R, Barnighausen T, et al. The global economic burden of diabetes in adults aged 20–79 years: a cost-of-illness study. Lancet Diabetes Endocrinol (2017) 5(6):423–30. doi:10.1016/S2213-8587(17)30097-9
PubMed Abstract | CrossRef Full Text | Google Scholar
6. Bommer C, Sagalova V, Heesemann E, Manne-Goehler J, Atun R, Barnighausen T, et al. Global economic burden of diabetes in adults: projections from 2015 to 2030. Diabetes Care (2018) 41(4):dc171962. doi:10.2337/dc17-1962
PubMed Abstract | CrossRef Full Text | Google Scholar
7. American Diabetes Association. Standards of medical care in diabetes-2018. Diabetes Care (2018) 41(Suppl 1):S152–3. doi:10.2337/dc18-S015
CrossRef Full Text | Google Scholar
8. Katzmarzyk PT, Lee IM, Martin CK, Blair SN. Epidemiology of physical activity and exercise training in the United States. Prog Cardiovasc Dis (2017) 60(1):3–10. doi:10.1016/j.pcad.2017.01.004
CrossRef Full Text | Google Scholar
9. Garber CE, Blissmer B, Deschenes MR, Franklin BA, Lamonte MJ, Lee IM, et al. American College of Sports Medicine position stand. Quantity and quality of exercise for developing and maintaining cardiorespiratory, musculoskeletal, and neuromotor fitness in apparently healthy adults: guidance for prescribing exercise. Med Sci Sports Exerc (2011) 43(7):1334–59. doi:10.1249/MSS.0b013e318213fefb
PubMed Abstract | CrossRef Full Text | Google Scholar
10. Sluik D, Buijsse B, Muckelbauer R, Kaaks R, Teucher B, Johnsen NF, et al. Physical activity and mortality in individuals with diabetes mellitus: a prospective study and meta-analysis. Arch Intern Med (2012) 172(17):1285–95. doi:10.1001/archinternmed.2012.3130
PubMed Abstract | CrossRef Full Text | Google Scholar
11. Wei M, Gibbons LW, Kampert JB, Nichaman MZ, Blair SN. Low cardiorespiratory fitness and physical inactivity as predictors of mortality in men with type 2 diabetes. Ann Intern Med (2000) 132(8):605–11. doi:10.7326/0003-4819-132-8-200004180-00035
PubMed Abstract | CrossRef Full Text | Google Scholar
12. Cooper AJ, Brage S, Ekelund U, Wareham NJ, Griffin SJ, Simmons RK. Association between objectively assessed sedentary time and physical activity with metabolic risk factors among people with recently diagnosed type 2 diabetes. Diabetologia (2014) 57(1):73–82. doi:10.1007/s00125-013-3069-8
PubMed Abstract | CrossRef Full Text | Google Scholar
13. de Borba AT, Jost RT, Gass R, Nedel FB, Cardoso DM, Pohl HH, et al. The influence of active and passive smoking on the cardiorespiratory fitness of adults. Multidiscip Respir Med (2014) 9(1):34. doi:10.1186/2049-6958-9-34
PubMed Abstract | CrossRef Full Text | Google Scholar
14. Mondal H, Mishra SP. Effect of BMI, body fat percentage and fat free mass on maximal oxygen consumption in healthy young adults. J Clin Diagn Res (2017) 11(6):CC17–20. doi:10.7860/JCDR/2017/25465.10039
PubMed Abstract | CrossRef Full Text | Google Scholar
15. Dumuid D, Lewis LK, Olds TS, Maher C, Bondarenko C, Norton L. Relationships between older adults’ use of time and cardio-respiratory fitness, obesity and cardio-metabolic risk: a compositional isotemporal substitution analysis. Maturitas (2018) 110:104–10. doi:10.1016/j.maturitas.2018.02.003
PubMed Abstract | CrossRef Full Text | Google Scholar
16. Yoon JH, So WY. Associations of hypertension status with physical fitness variables in Korean women. Iran J Public Health (2013) 42(7):673–80.
PubMed Abstract | Google Scholar
17. Mazic S, Suzic Lazic J, Dekleva M, Antic M, Soldatovic I, Djelic M, et al. The impact of elevated blood pressure on exercise capacity in elite athletes. Int J Cardiol (2015) 180:171–7. doi:10.1016/j.ijcard.2014.11.125
PubMed Abstract | CrossRef Full Text | Google Scholar
18. Jae SY, Kurl S, Franklin BA, Laukkanen JA. Changes in cardiorespiratory fitness predict incident hypertension: a population-based long-term study. Am J Hum Biol (2017) 29(3):e22932. doi:10.1002/ajhb.22932
PubMed Abstract | CrossRef Full Text | Google Scholar
19. Regensteiner JG, Bauer TA, Reusch JE, Quaife RA, Chen MY, Smith SC, et al. Cardiac dysfunction during exercise in uncomplicated type 2 diabetes. Med Sci Sports Exerc (2009) 41(5):977–84. doi:10.1249/MSS.0b013e3181942051
PubMed Abstract | CrossRef Full Text | Google Scholar
20. Regensteiner JG, Sippel J, McFarling ET, Wolfel EE, Hiatt WR. Effects of non-insulin-dependent diabetes on oxygen consumption during treadmill exercise. Med Sci Sports Exerc (1995) 27(6):875–81. doi:10.1249/00005768-199505000-00007
PubMed Abstract | CrossRef Full Text | Google Scholar
21. Brandenburg SL, Reusch JE, Bauer TA, Jeffers BW, Hiatt WR, Regensteiner JG. Effects of exercise training on oxygen uptake kinetic responses in women with type 2 diabetes. Diabetes Care (1999) 22(10):1640–6. doi:10.2337/diacare.22.10.1640
PubMed Abstract | CrossRef Full Text | Google Scholar
22. Bauer TA, Reusch JE, Levi M, Regensteiner JG. Skeletal muscle deoxygenation following the onset of moderate exercise suggests slowed microvascular blood flow kinetics in type 2 diabetes. Diabetes Care (2007) 30(11):2880–5. doi:10.2337/dc07-0843
CrossRef Full Text | Google Scholar
23. Nadeau KJ, Zeitler PS, Bauer TA, Brown MS, Dorosz JL, Draznin B, et al. Insulin resistance in adolescents with type 2 diabetes is associated with impaired exercise capacity. J Clin Endocrinol Metab (2009) 94(10):3687–95. doi:10.1210/jc.2008-2844
PubMed Abstract | CrossRef Full Text | Google Scholar
24. Nadeau KJ, Regensteiner JG, Bauer TA, Brown MS, Dorosz JL, Hull A, et al. Insulin resistance in adolescents with type 1 diabetes and its relationship to cardiovascular function. J Clin Endocrinol Metab (2010) 95(2):513–21. doi:10.1210/jc.2009-1756
PubMed Abstract | CrossRef Full Text | Google Scholar
25. Ross R, Blair SN, Arena R, Church TS, Despres JP, Franklin BA, et al. Importance of assessing cardiorespiratory fitness in clinical practice: a case for fitness as a clinical vital sign: a scientific statement from the American Heart Association. Circulation (2016) 134(24):e653–99. doi:10.1161/CIR.0000000000000461
PubMed Abstract | CrossRef Full Text | Google Scholar
26. Lee CD, Blair SN, Jackson AS. Cardiorespiratory fitness, body composition, and all-cause and cardiovascular disease mortality in men. Am J Clin Nutr (1999) 69(3):373–80. doi:10.1093/ajcn/69.3.373
PubMed Abstract | CrossRef Full Text | Google Scholar
27. Wei M, Kampert JB, Barlow CE, Nichaman MZ, Gibbons LW, Paffenbarger RS Jr, et al. Relationship between low cardiorespiratory fitness and mortality in normal-weight, overweight, and obese men. JAMA (1999) 282(16):1547–53. doi:10.1001/jama.282.16.1547
PubMed Abstract | CrossRef Full Text | Google Scholar
28. Weintraub WS, Daniels SR, Burke LE, Franklin BA, Goff DC Jr, Hayman LL, et al. Value of primordial and primary prevention for cardiovascular disease: a policy statement from the American Heart Association. Circulation (2011) 124(8):967–90. doi:10.1161/CIR.0b013e3182285a81
PubMed Abstract | CrossRef Full Text | Google Scholar
29. Regensteiner JG, Bauer TA, Reusch JE, Brandenburg SL, Sippel JM, Vogelsong AM, et al. Abnormal oxygen uptake kinetic responses in women with type II diabetes mellitus. J Appl Physiol (1985) (1998) 85(1):310–7. doi:10.1152/jappl.1998.85.1.310
PubMed Abstract | CrossRef Full Text | Google Scholar
30. Huebschmann AG, Reis EN, Emsermann C, Dickinson LM, Reusch JE, Bauer TA, et al. Women with type 2 diabetes perceive harder effort during exercise than nondiabetic women. Appl Physiol Nutr Metab (2009) 34(5):851–7. doi:10.1139/H09-074
PubMed Abstract | CrossRef Full Text | Google Scholar
31. Regensteiner JG, Bauer TA, Reusch JE. Rosiglitazone improves exercise capacity in individuals with type 2 diabetes. Diabetes Care (2005) 28(12):2877–83. doi:10.2337/diacare.28.12.2877
PubMed Abstract | CrossRef Full Text | Google Scholar
32. Subudhi AW, Dimmen AC, Roach RC. Effects of acute hypoxia on cerebral and muscle oxygenation during incremental exercise. J Appl Physiol (1985) (2007) 103(1):177–83. doi:10.1152/japplphysiol.01460.2006
CrossRef Full Text | Google Scholar
33. MacAnaney O, Reilly H, O’Shea D, Egana M, Green S. Effect of type 2 diabetes on the dynamic response characteristics of leg vascular conductance during exercise. Diab Vasc Dis Res (2011) 8(1):12–21. doi:10.1177/1479164110389625
PubMed Abstract | CrossRef Full Text | Google Scholar
34. Jones WS, Duscha BD, Robbins JL, Duggan NN, Regensteiner JG, Kraus WE, et al. Alteration in angiogenic and anti-angiogenic forms of vascular endothelial growth factor-A in skeletal muscle of patients with intermittent claudication following exercise training. Vasc Med (2012) 17(2):94–100. doi:10.1177/1358863X11436334
PubMed Abstract | CrossRef Full Text | Google Scholar
35. Macananey O, O’Shea D, Warmington SA, Green S, Egana M. Gymnasium-based unsupervised exercise maintains benefits in oxygen uptake kinetics obtained following supervised training in type 2 diabetes. Appl Physiol Nutr Metab (2012) 37(4):599–609. doi:10.1139/h2012-012
PubMed Abstract | CrossRef Full Text | Google Scholar
36. Huebschmann AG, Kohrt WM, Herlache L, Wolfe P, Daugherty S, Reusch JE, et al. Type 2 diabetes exaggerates exercise effort and impairs exercise performance in older women. BMJ Open Diabetes Res Care (2015) 3(1):e000124. doi:10.1136/bmjdrc-2015-000124
PubMed Abstract | CrossRef Full Text | Google Scholar
37. Schneider SH, Amorosa LF, Khachadurian AK, Ruderman NB. Studies on the mechanism of improved glucose control during regular exercise in type 2 (non-insulin-dependent) diabetes. Diabetologia (1984) 26(5):355–60. doi:10.1007/BF00266036
PubMed Abstract | CrossRef Full Text | Google Scholar
38. Kjaer M, Hollenbeck CB, Frey-Hewitt B, Galbo H, Haskell W, Reaven GM. Glucoregulation and hormonal responses to maximal exercise in non-insulin-dependent diabetes. J Appl Physiol (1985) (1990) 68(5):2067–74. doi:10.1152/jappl.1990.68.5.2067
PubMed Abstract | CrossRef Full Text | Google Scholar
39. Mac Ananey O, Malone J, Warmington S, O’Shea D, Green S, Egana M. Cardiac output is not related to the slowed O2 uptake kinetics in type 2 diabetes. Med Sci Sports Exerc (2011) 43(6):935–42. doi:10.1249/MSS.0b013e3182061cdb
PubMed Abstract | CrossRef Full Text | Google Scholar
40. Gusso S, Hofman P, Lalande S, Cutfield W, Robinson E, Baldi JC. Impaired stroke volume and aerobic capacity in female adolescents with type 1 and type 2 diabetes mellitus. Diabetologia (2008) 51(7):1317–20. doi:10.1007/s00125-008-1012-1
PubMed Abstract | CrossRef Full Text | Google Scholar
41. Wilkerson DP, Poole DC, Jones AM, Fulford J, Mawson DM, Ball CI, et al. Older type 2 diabetic males do not exhibit abnormal pulmonary oxygen uptake and muscle oxygen utilization dynamics during submaximal cycling exercise. Am J Physiol Regul Integr Comp Physiol (2011) 300(3):R685–92. doi:10.1152/ajpregu.00479.2010
CrossRef Full Text | Google Scholar
42. Hamman RF, Wing RR, Edelstein SL, Lachin JM, Bray GA, Delahanty L, et al. Effect of weight loss with lifestyle intervention on risk of diabetes. Diabetes Care (2006) 29(9):2102–7. doi:10.2337/dc06-0560
PubMed Abstract | CrossRef Full Text | Google Scholar
43. Bouchard C, Leon AS, Rao DC, Skinner JS, Wilmore JH, Gagnon J. The HERITAGE family study. Aims, design, and measurement protocol. Med Sci Sports Exerc (1995) 27(5):721–9. doi:10.1249/00005768-199505000-00015
PubMed Abstract | CrossRef Full Text | Google Scholar
44. Morss GM, Jordan AN, Skinner JS, Dunn AL, Church TS, Earnest CP, et al. Dose response to exercise in women aged 45–75 yr (DREW): design and rationale. Med Sci Sports Exerc (2004) 36(2):336–44. doi:10.1249/01.MSS.0000113738.06267.E5
PubMed Abstract | CrossRef Full Text | Google Scholar
45. Slentz CA, Aiken LB, Houmard JA, Bales CW, Johnson JL, Tanner CJ, et al. Inactivity, exercise, and visceral fat. STRRIDE: a randomized, controlled study of exercise intensity and amount. J Appl Physiol (1985) (2005) 99(4):1613–8. doi:10.1152/japplphysiol.00124.2005
PubMed Abstract | CrossRef Full Text | Google Scholar
46. Thompson AM, Mikus CR, Rodarte RQ, Distefano B, Priest EL, Sinclair E, et al. Inflammation and exercise (INFLAME): study rationale, design, and methods. Contemp Clin Trials (2008) 29(3):418–27. doi:10.1016/j.cct.2007.09.009
PubMed Abstract | CrossRef Full Text | Google Scholar
47. Sisson SB, Katzmarzyk PT, Earnest CP, Bouchard C, Blair SN, Church TS. Volume of exercise and fitness nonresponse in sedentary, postmenopausal women. Med Sci Sports Exerc (2009) 41(3):539–45. doi:10.1249/MSS.0b013e3181896c4e
PubMed Abstract | CrossRef Full Text | Google Scholar
48. Regensteiner JG, Bauer TA, Huebschmann AG, Herlache L, Weinberger HD, Wolfel EE, et al. Sex differences in the effects of type 2 diabetes on exercise performance. Med Sci Sports Exerc (2015) 47(1):58–65. doi:10.1249/MSS.0000000000000371
PubMed Abstract | CrossRef Full Text | Google Scholar
49. Ring M, Eriksson MJ, Fritz T, Nyberg G, Ostenson CG, Krook A, et al. Influence of physical activity and gender on arterial function in type 2 diabetes, normal and impaired glucose tolerance. Diab Vasc Dis Res (2015) 12(5):315–24. doi:10.1177/1479164115588548
PubMed Abstract | CrossRef Full Text | Google Scholar
50. Look ARG, Wing RR, Bolin P, Brancati FL, Bray GA, Clark JM, et al. Cardiovascular effects of intensive lifestyle intervention in type 2 diabetes. N Engl J Med (2013) 369(2):145–54. doi:10.1056/NEJMoa1212914
PubMed Abstract | CrossRef Full Text | Google Scholar
51. Pi-Sunyer X. The look AHEAD trial: a review and discussion of its outcomes. Curr Nutr Rep (2014) 3(4):387–91. doi:10.1007/s13668-014-0099-x
PubMed Abstract | CrossRef Full Text | Google Scholar
52. Kadoglou NP, Iliadis F, Sailer N, Athanasiadou Z, Vitta I, Kapelouzou A, et al. Exercise training ameliorates the effects of rosiglitazone on traditional and novel cardiovascular risk factors in patients with type 2 diabetes mellitus. Metabolism (2010) 59(4):599–607. doi:10.1016/j.metabol.2009.09.002
PubMed Abstract | CrossRef Full Text | Google Scholar
53. Kim JC. The effect of exercise training combined with PPARgamma agonist on skeletal muscle glucose uptake and insulin sensitivity in induced diabetic obese Zucker rats. J Exerc Nutrition Biochem (2016) 20(2):42–50. doi:10.20463/jenb.2016.06.20.2.6
CrossRef Full Text | Google Scholar
54. McGuire DK, Abdullah SM, See R, Snell PG, McGavock J, Szczepaniak LS, et al. Randomized comparison of the effects of rosiglitazone vs. placebo on peak integrated cardiovascular performance, cardiac structure, and function. Eur Heart J (2010) 31(18):2262–70. doi:10.1093/eurheartj/ehq228
PubMed Abstract | CrossRef Full Text | Google Scholar
55. Hiatt WR, Kaul S, Smith RJ. The cardiovascular safety of diabetes drugs—insights from the rosiglitazone experience. N Engl J Med (2013) 369(14):1285–7. doi:10.1056/NEJMp1309610
CrossRef Full Text | Google Scholar
56. Braun B, Eze P, Stephens BR, Hagobian TA, Sharoff CG, Chipkin SR, et al. Impact of metformin on peak aerobic capacity. Appl Physiol Nutr Metab (2008) 33(1):61–7. doi:10.1139/H07-144
PubMed Abstract | CrossRef Full Text | Google Scholar
57. Cadeddu C, Nocco S, Deidda M, Cadeddu F, Bina A, Demuru P, et al. Cardiopulmonary and endothelial effects of metformin treatment in an insulin resistant population. Int J Cardiol (2012) 158(2):302–4. doi:10.1016/j.ijcard.2012.04.113
CrossRef Full Text | Google Scholar
58. Paul AA, Dkhar SA, Kamalanathan S, Thabah MM, George M, Chandrasekaran I, et al. Effect of metformin on exercise capacity in metabolic syndrome. Diabetes Metab Syndr (2017) 11(Suppl 1):S403–6. doi:10.1016/j.dsx.2017.03.025
PubMed Abstract | CrossRef Full Text | Google Scholar
59. Malin SK, Gerber R, Chipkin SR, Braun B. Independent and combined effects of exercise training and metformin on insulin sensitivity in individuals with prediabetes. Diabetes Care (2012) 35(1):131–6. doi:10.2337/dc11-0925
PubMed Abstract | CrossRef Full Text | Google Scholar
60. Cadeddu C, Nocco S, Lucia C, Deidda M, Bina A, Fabio O, et al. Effects of metformin and exercise training, alone or in association, on cardio-pulmonary performance and quality of life in insulin resistance patients. Cardiovasc Diabetol (2014) 13:93. doi:10.1186/1475-2840-13-93
PubMed Abstract | CrossRef Full Text | Google Scholar
61. Leverve XM, Guigas B, Detaille D, Batandier C, Koceir EA, Chauvin C, et al. Mitochondrial metabolism and type-2 diabetes: a specific target of metformin. Diabetes Metab (2003) 29(4 Pt 2):S88–94. doi:10.1016/S1262-3636(03)72792-X
PubMed Abstract | CrossRef Full Text | Google Scholar
62. Batandier C, Guigas B, Detaille D, El-Mir MY, Fontaine E, Rigoulet M, et al. The ROS production induced by a reverse-electron flux at respiratory-chain complex 1 is hampered by metformin. J Bioenerg Biomembr (2006) 38(1):33–42. doi:10.1007/s10863-006-9003-8
PubMed Abstract | CrossRef Full Text | Google Scholar
63. Barres R, Osler ME, Yan J, Rune A, Fritz T, Caidahl K, et al. Non-CpG methylation of the PGC-1alpha promoter through DNMT3B controls mitochondrial density. Cell Metab (2009) 10(3):189–98. doi:10.1016/j.cmet.2009.07.011
PubMed Abstract | CrossRef Full Text | Google Scholar
64. Barres R, Yan J, Egan B, Treebak JT, Rasmussen M, Fritz T, et al. Acute exercise remodels promoter methylation in human skeletal muscle. Cell Metab (2012) 15(3):405–11. doi:10.1016/j.cmet.2012.01.001
PubMed Abstract | CrossRef Full Text | Google Scholar
65. Sparks LM. Exercise training response heterogeneity: physiological and molecular insights. Diabetologia (2017) 60(12):2329–36. doi:10.1007/s00125-017-4461-6
PubMed Abstract | CrossRef Full Text | Google Scholar
66. Kelley DE, He J, Menshikova EV, Ritov VB. Dysfunction of mitochondria in human skeletal muscle in type 2 diabetes. Diabetes (2002) 51(10):2944–50. doi:10.2337/diabetes.51.10.2944
PubMed Abstract | CrossRef Full Text | Google Scholar
67. Bugger H, Abel ED. Mitochondria in the diabetic heart. Cardiovasc Res (2010) 88(2):229–40. doi:10.1093/cvr/cvq239
PubMed Abstract | CrossRef Full Text | Google Scholar
68. Knaub LA, McCune S, Chicco AJ, Miller M, Moore RL, Birdsey N, et al. Impaired response to exercise intervention in the vasculature in metabolic syndrome. Diab Vasc Dis Res (2013) 10(3):222–38. doi:10.1177/1479164112459664
PubMed Abstract | CrossRef Full Text | Google Scholar
69. Davidson SM. Endothelial mitochondria and heart disease. Cardiovasc Res (2010) 88(1):58–66. doi:10.1093/cvr/cvq195
CrossRef Full Text | Google Scholar
70. Keller AC, Knaub LA, Miller MW, Birdsey N, Klemm DJ, Reusch JE. Saxagliptin restores vascular mitochondrial exercise response in the Goto-Kakizaki rat. J Cardiovasc Pharmacol (2015) 65(2):137–47. doi:10.1097/FJC.0000000000000170
PubMed Abstract | CrossRef Full Text | Google Scholar
71. Keller AC, Knaub LA, McClatchey PM, Connon CA, Bouchard R, Miller MW, et al. Differential mitochondrial adaptation in primary vascular smooth muscle cells from a diabetic rat model. Oxid Med Cell Longev (2016) 2016:8524267. doi:10.1155/2016/8524267
PubMed Abstract | CrossRef Full Text | Google Scholar
72. Brierley EJ, Johnson MA, James OF, Turnbull DM. Effects of physical activity and age on mitochondrial function. QJM (1996) 89(4):251–8. doi:10.1093/qjmed/89.4.251
PubMed Abstract | CrossRef Full Text | Google Scholar
73. Spina RJ, Chi MM, Hopkins MG, Nemeth PM, Lowry OH, Holloszy JO. Mitochondrial enzymes increase in muscle in response to 7–10 days of cycle exercise. J Appl Physiol (1985) (1996) 80(6):2250–4. doi:10.1152/jappl.1996.80.6.2250
PubMed Abstract | CrossRef Full Text | Google Scholar
74. Starritt EC, Angus D, Hargreaves M. Effect of short-term training on mitochondrial ATP production rate in human skeletal muscle. J Appl Physiol (1985) (1999) 86(2):450–4. doi:10.1152/jappl.1999.86.2.450
CrossRef Full Text | Google Scholar
75. Ji LL. Exercise-induced modulation of antioxidant defense. Ann N Y Acad Sci (2002) 959:82–92. doi:10.1111/j.1749-6632.2002.tb02085.x
PubMed Abstract | CrossRef Full Text | Google Scholar
76. Young CG, Knight CA, Vickers KC, Westbrook D, Madamanchi NR, Runge MS, et al. Differential effects of exercise on aortic mitochondria. Am J Physiol Heart Circ Physiol (2005) 288(4):H1683–9. doi:10.1152/ajpheart.00136.2004
PubMed Abstract | CrossRef Full Text | Google Scholar
77. Montagnani M, Chen H, Barr VA, Quon MJ. Insulin-stimulated activation of eNOS is independent of Ca2+ but requires phosphorylation by Akt at Ser(1179). J Biol Chem (2001) 276(32):30392–8. doi:10.1074/jbc.M103702200
PubMed Abstract | CrossRef Full Text | Google Scholar
78. Nisoli E, Clementi E, Paolucci C, Cozzi V, Tonello C, Sciorati C, et al. Mitochondrial biogenesis in mammals: the role of endogenous nitric oxide. Science (2003) 299(5608):896–9. doi:10.1126/science.1079368
PubMed Abstract | CrossRef Full Text | Google Scholar
79. Miller MW, Knaub LA, Olivera-Fragoso LF, Keller AC, Balasubramaniam V, Watson PA, et al. Nitric oxide regulates vascular adaptive mitochondrial dynamics. Am J Physiol Heart Circ Physiol (2013) 304(12):H1624–33. doi:10.1152/ajpheart.00987.2012
PubMed Abstract | CrossRef Full Text | Google Scholar
80. Krotkiewski M, Lonnroth P, Mandroukas K, Wroblewski Z, Rebuffe-Scrive M, Holm G, et al. The effects of physical training on insulin secretion and effectiveness and on glucose metabolism in obesity and type 2 (non-insulin-dependent) diabetes mellitus. Diabetologia (1985) 28(12):881–90. doi:10.1007/BF00703130
PubMed Abstract | CrossRef Full Text | Google Scholar
81. Kirwan JP, Kohrt WM, Wojta DM, Bourey RE, Holloszy JO. Endurance exercise training reduces glucose-stimulated insulin levels in 60- to 70-year-old men and women. J Gerontol (1993) 48(3):M84–90. doi:10.1093/geronj/48.3.M84
PubMed Abstract | CrossRef Full Text | Google Scholar
82. Marliss EB, Vranic M. Intense exercise has unique effects on both insulin release and its roles in glucoregulation: implications for diabetes. Diabetes (2002) 51(Suppl 1):S271–83. doi:10.2337/diabetes.51.2007.S271
PubMed Abstract | CrossRef Full Text | Google Scholar
83. Kiraly MA, Bates HE, Kaniuk NA, Yue JT, Brumell JH, Matthews SG, et al. Swim training prevents hyperglycemia in ZDF rats: mechanisms involved in the partial maintenance of beta-cell function. Am J Physiol Endocrinol Metab (2008) 294(2):E271–83. doi:10.1152/ajpendo.00476.2007
PubMed Abstract | CrossRef Full Text | Google Scholar
84. Park S, Hong SM, Sung SR. Exendin-4 and exercise promotes beta-cell function and mass through IRS2 induction in islets of diabetic rats. Life Sci (2008) 82(9–10):503–11. doi:10.1016/j.lfs.2007.12.018
PubMed Abstract | CrossRef Full Text | Google Scholar
85. DeFronzo RA. Glucose intolerance and aging. Diabetes Care (1981) 4(4):493–501. doi:10.2337/diacare.4.4.493
CrossRef Full Text | Google Scholar
86. Fink RI, Kolterman OG, Griffin J, Olefsky JM. Mechanisms of insulin resistance in aging. J Clin Invest (1983) 71(6):1523–35. doi:10.1172/JCI110908
CrossRef Full Text | Google Scholar
87. Iozzo P, Beck-Nielsen H, Laakso M, Smith U, Yki-Jarvinen H, Ferrannini E. Independent influence of age on basal insulin secretion in nondiabetic humans. European Group for the Study of Insulin Resistance. J Clin Endocrinol Metab (1999) 84(3):863–8. doi:10.1210/jcem.84.3.5542
PubMed Abstract | CrossRef Full Text | Google Scholar
88. Szoke E, Shrayyef MZ, Messing S, Woerle HJ, van Haeften TW, Meyer C, et al. Effect of aging on glucose homeostasis: accelerated deterioration of beta-cell function in individuals with impaired glucose tolerance. Diabetes Care (2008) 31(3):539–43. doi:10.2337/dc07-1443
PubMed Abstract | CrossRef Full Text | Google Scholar
89. De Tata V. Age-related impairment of pancreatic beta-cell function: pathophysiological and cellular mechanisms. Front Endocrinol (2014) 5:138. doi:10.3389/fendo.2014.00138
PubMed Abstract | CrossRef Full Text | Google Scholar
90. Santulli G, Lombardi A, Sorriento D, Anastasio A, Del Giudice C, Formisano P, et al. Age-related impairment in insulin release: the essential role of beta(2)-adrenergic receptor. Diabetes (2012) 61(3):692–701. doi:10.2337/db11-1027
CrossRef Full Text | Google Scholar
91. Lin Y, Sun Z. Antiaging gene Klotho enhances glucose-induced insulin secretion by up-regulating plasma membrane levels of TRPV2 in MIN6 beta-cells. Endocrinology (2012) 153(7):3029–39. doi:10.1210/en.2012-1091
CrossRef Full Text | Google Scholar
92. Bonomini F, Rodella LF, Rezzani R. Metabolic syndrome, aging and involvement of oxidative stress. Aging Dis (2015) 6(2):109–20. doi:10.14336/AD.2014.0305
PubMed Abstract | CrossRef Full Text | Google Scholar
93. Rogers MA, Hagberg JM, Martin WH III, Ehsani AA, Holloszy JO. Decline in VO2max with aging in master athletes and sedentary men. J Appl Physiol (1985) (1990) 68(5):2195–9. doi:10.1152/jappl.1990.68.5.2195
PubMed Abstract | CrossRef Full Text | Google Scholar
94. Ishihara H, Maechler P, Gjinovci A, Herrera PL, Wollheim CB. Islet beta-cell secretion determines glucagon release from neighbouring alpha-cells. Nat Cell Biol (2003) 5(4):330–5. doi:10.1038/ncb951
PubMed Abstract | CrossRef Full Text | Google Scholar
95. Lowell BB, Shulman GI. Mitochondrial dysfunction and type 2 diabetes. Science (2005) 307(5708):384–7. doi:10.1126/science.1104343
CrossRef Full Text | Google Scholar
96. Lu H, Koshkin V, Allister EM, Gyulkhandanyan AV, Wheeler MB. Molecular and metabolic evidence for mitochondrial defects associated with beta-cell dysfunction in a mouse model of type 2 diabetes. Diabetes (2010) 59(2):448–59. doi:10.2337/db09-0129
PubMed Abstract | CrossRef Full Text | Google Scholar
97. Lombardi A, Trimarco B, Iaccarino G, Santulli G. Impaired mitochondrial calcium uptake caused by tacrolimus underlies beta-cell failure. Cell Commun Signal (2017) 15(1):47. doi:10.1186/s12964-017-0203-0
PubMed Abstract | CrossRef Full Text | Google Scholar
98. Gros R, You X, Baggio LL, Kabir MG, Sadi AM, Mungrue IN, et al. Cardiac function in mice lacking the glucagon-like peptide-1 receptor. Endocrinology (2003) 144(6):2242–52. doi:10.1210/en.2003-0007
PubMed Abstract | CrossRef Full Text | Google Scholar
99. Ban K, Noyan-Ashraf MH, Hoefer J, Bolz SS, Drucker DJ, Husain M. Cardioprotective and vasodilatory actions of glucagon-like peptide 1 receptor are mediated through both glucagon-like peptide 1 receptor-dependent and -independent pathways. Circulation (2008) 117(18):2340–50. doi:10.1161/CIRCULATIONAHA.107.739938
PubMed Abstract | CrossRef Full Text | Google Scholar
100. Chai W, Dong Z, Wang N, Wang W, Tao L, Cao W, et al. Glucagon-like peptide 1 recruits microvasculature and increases glucose use in muscle via a nitric oxide-dependent mechanism. Diabetes (2012) 61(4):888–96. doi:10.2337/db11-1073
PubMed Abstract | CrossRef Full Text | Google Scholar
101. Dong Z, Chai W, Wang W, Zhao L, Fu Z, Cao W, et al. Protein kinase A mediates glucagon-like peptide 1-induced nitric oxide production and muscle microvascular recruitment. Am J Physiol Endocrinol Metab (2013) 304(2):E222–8. doi:10.1152/ajpendo.00473.2012
PubMed Abstract | CrossRef Full Text | Google Scholar
102. Scalzo RL, Moreau KL, Ozemek C, Herlache L, McMillin S, Gilligan S, et al. Exenatide improves diastolic function and attenuates arterial stiffness but does not alter exercise capacity in individuals with type 2 diabetes. J Diabetes Complications (2017) 31(2):449–55. doi:10.1016/j.jdiacomp.2016.10.003
PubMed Abstract | CrossRef Full Text | Google Scholar
103. Narkar VA, Downes M, Yu RT, Embler E, Wang YX, Banayo E, et al. AMPK and PPARdelta agonists are exercise mimetics. Cell (2008) 134(3):405–15. doi:10.1016/j.cell.2008.06.051
PubMed Abstract | CrossRef Full Text | Google Scholar
104. Fan W, Waizenegger W, Lin CS, Sorrentino V, He MX, Wall CE, et al. PPARdelta promotes running endurance by preserving glucose. Cell Metab (2017) 25(5):1186–93.e4. doi:10.1016/j.cmet.2017.04.006
CrossRef Full Text | Google Scholar
105. Zinman B, Wanner C, Lachin JM, Fitchett D, Bluhmki E, Hantel S, et al. Empagliflozin, cardiovascular outcomes, and mortality in type 2 diabetes. N Engl J Med (2015) 373(22):2117–28. doi:10.1056/NEJMoa1504720
CrossRef Full Text | Google Scholar
106. Neal B, Perkovic V, Mahaffey KW, de Zeeuw D, Fulcher G, Erondu N, et al. Canagliflozin and cardiovascular and renal events in type 2 diabetes. N Engl J Med (2017) 377(7):644–57. doi:10.1056/NEJMoa1611925
CrossRef Full Text | Google Scholar
107. O’Brien TP, Jenkins EC, Estes SK, Castaneda AV, Ueta K, Farmer TD, et al. Correcting postprandial hyperglycemia in Zucker diabetic fatty rats with an SGLT2 inhibitor restores glucose effectiveness in the liver and reduces insulin resistance in skeletal muscle. Diabetes (2017) 66(5):1172–84. doi:10.2337/db16-1410
PubMed Abstract | CrossRef Full Text | Google Scholar
108. Diaz-Rodriguez E, Agra RM, Fernandez AL, Adrio B, Garcia-Caballero T, Gonzalez-Juanatey JR, et al. Effects of dapagliflozin on human epicardial adipose tissue: modulation of insulin resistance, inflammatory chemokine production, and differentiation ability. Cardiovasc Res (2018) 114(2):336–46. doi:10.1093/cvr/cvx186
PubMed Abstract | CrossRef Full Text | Google Scholar
109. Li CY, Wang LX, Dong SS, Hong Y, Zhou XH, Zheng WW, et al. Phlorizin exerts direct protective effects on palmitic acid (PA)-induced endothelial dysfunction by activating the PI3K/AKT/eNOS signaling pathway and increasing the levels of nitric oxide (NO). Med Sci Monit Basic Res (2018) 24:1–9. doi:10.12659/MSMBR.907775
PubMed Abstract | CrossRef Full Text | Google Scholar
110. Radholm K, Figtree G, Perkovic V, Solomon SD, Mahaffey KW, de Zeeuw D, et al. Canagliflozin and heart failure in type 2 diabetes mellitus: results from the CANVAS program (Canagliflozin Cardiovascular Assessment Study). Circulation (2018). doi:10.1161/CIRCULATIONAHA.118.034222
CrossRef Full Text | Google Scholar
111. Cherney DZ, Perkins BA, Soleymanlou N, Har R, Fagan N, Johansen OE, et al. The effect of empagliflozin on arterial stiffness and heart rate variability in subjects with uncomplicated type 1 diabetes mellitus. Cardiovasc Diabetol (2014) 13:28. doi:10.1186/1475-2840-13-28
PubMed Abstract | CrossRef Full Text | Google Scholar
112. Chilton R, Tikkanen I, Cannon CP, Crowe S, Woerle HJ, Broedl UC, et al. Effects of empagliflozin on blood pressure and markers of arterial stiffness and vascular resistance in patients with type 2 diabetes. Diabetes Obes Metab (2015) 17(12):1180–93. doi:10.1111/dom.12572
PubMed Abstract | CrossRef Full Text | Google Scholar
113. Fioretto P, Zambon A, Rossato M, Busetto L, Vettor R. SGLT2 inhibitors and the diabetic kidney. Diabetes Care (2016) 39(Suppl 2):S165–71. doi:10.2337/dcS15-3006
PubMed Abstract | CrossRef Full Text | Google Scholar
114. Wanner C, Inzucchi SE, Lachin JM, Fitchett D, von Eynatten M, Mattheus M, et al. Empagliflozin and progression of kidney disease in type 2 diabetes. N Engl J Med (2016) 375(4):323–34. doi:10.1056/NEJMoa1515920
CrossRef Full Text | Google Scholar
115. Habibi J, Aroor AR, Sowers JR, Jia G, Hayden MR, Garro M, et al. Sodium glucose transporter 2 (SGLT2) inhibition with empagliflozin improves cardiac diastolic function in a female rodent model of diabetes. Cardiovasc Diabetol (2017) 16(1):9. doi:10.1186/s12933-016-0489-z
PubMed Abstract | CrossRef Full Text | Google Scholar
116. Packer M, Anker SD, Butler J, Filippatos G, Zannad F. Effects of sodium-glucose cotransporter 2 inhibitors for the treatment of patients with heart failure: proposal of a novel mechanism of action. JAMA Cardiol (2017) 2(9):1025–9. doi:10.1001/jamacardio.2017.2275
PubMed Abstract | CrossRef Full Text | Google Scholar
117. Wadley GD, Choate J, McConell GK. NOS isoform-specific regulation of basal but not exercise-induced mitochondrial biogenesis in mouse skeletal muscle. J Physiol (2007) 585(Pt 1):253–62. doi:10.1113/jphysiol.2007.141309
CrossRef Full Text | Google Scholar
118. Sjoberg KA, Frosig C, Kjobsted R, Sylow L, Kleinert M, Betik AC, et al. Exercise increases human skeletal muscle insulin sensitivity via coordinated increases in microvascular perfusion and molecular signaling. Diabetes (2017) 66(6):1501–10. doi:10.2337/db16-1327
PubMed Abstract | CrossRef Full Text | Google Scholar
119. Barrett EJ, Eggleston EM, Inyard AC, Wang H, Li G, Chai W, et al. The vascular actions of insulin control its delivery to muscle and regulate the rate-limiting step in skeletal muscle insulin action. Diabetologia (2009) 52(5):752–64. doi:10.1007/s00125-009-1313-z
PubMed Abstract | CrossRef Full Text | Google Scholar
120. Wang H, Wang AX, Aylor K, Barrett EJ. Nitric oxide directly promotes vascular endothelial insulin transport. Diabetes (2013) 62(12):4030–42. doi:10.2337/db13-0627
PubMed Abstract | CrossRef Full Text | Google Scholar
121. Mason McClatchey P, Bauer TA, Regensteiner JG, Schauer IE, Huebschmann AG, Reusch JEB. Dissociation of local and global skeletal muscle oxygen transport metrics in type 2 diabetes. J Diabetes Complications (2017) 31(8):1311–7. doi:10.1016/j.jdiacomp.2017.05.004
PubMed Abstract | CrossRef Full Text | Google Scholar
122. McClatchey PM, Frisbee JC, Reusch JEB. A conceptual framework for predicting and addressing the consequences of disease-related microvascular dysfunction. Microcirculation (2017) 24(6):e12359. doi:10.1111/micc.12359
PubMed Abstract | CrossRef Full Text | Google Scholar
123. Mason McClatchey P, Wu F, Olfert IM, Ellis CG, Goldman D, Reusch JE, et al. Impaired tissue oxygenation in metabolic syndrome requires increased microvascular perfusion heterogeneity. J Cardiovasc Transl Res (2017) 10(1):69–81. doi:10.1007/s12265-017-9732-6